News
Welcome to Arab Health 2023!
Meet RUMEX at the greatest event in Dubai, UAE!
We welcome you to visit RUMEX booth at the Arab Health 2023 exhibition.
Dates: January 30 - February 2, 2023
Place: Dubai World Trade Centre - Trade Centre 2
Find us: Hall 1, USA Pavilion, RUMEX booth H1.B35
We would be delighted to present our product portfolio and effective solutions for turnkey projects.
Have a look at instruments and make sure of their indisputable quality!
See lessHoliday Schedule
Customer Service Department and Warehouse will be closed on the following days due to the holiday observances:
December 23 — 26, 2022 (Christmas Holidays);
December 30, 2022— January 2, 2023 (New Year Holidays);
January 3 — 11, 2023 (Annual Inventory).
Please let some time for your orders to be shipped. Send us all inquiries by email and we will reply as soon as the office is open.
RUMEX at the Joint XLIV Inter-American Course in Clinical Ophthalmology and XXVI Pan-American Regional Course 2022
We were more than happy to see our dear partners and customers at the Joint XLIV Inter-American Course in Clinical Ophthalmology and XXVI Pan-American Regional Course in Miami the last weekend.
See some photos below.


RUMEX at AAO 2022
RUMEX team took part in AAO 2022 in Chicago this October. We were happy to see our dear partners and customers and meet their demands in high quality instruments and consumables. Thanks to American Academy of Ophthalmology for the well-organized exhibition and congress. RUMEX is happy to be the part of this event as a reliable partner for many years.
See some photos below.



RUMEX at ESCRS 2022
We had a great time at ESCRS 2022 which was held on September 16-19, 2022 in Milan.
RUMEX team thanks everyone who came to visit our booth.
We were glad to present new items, special offers and discounts to our dear customers.
Please see some photos below
Hope to meet you at the coming events.
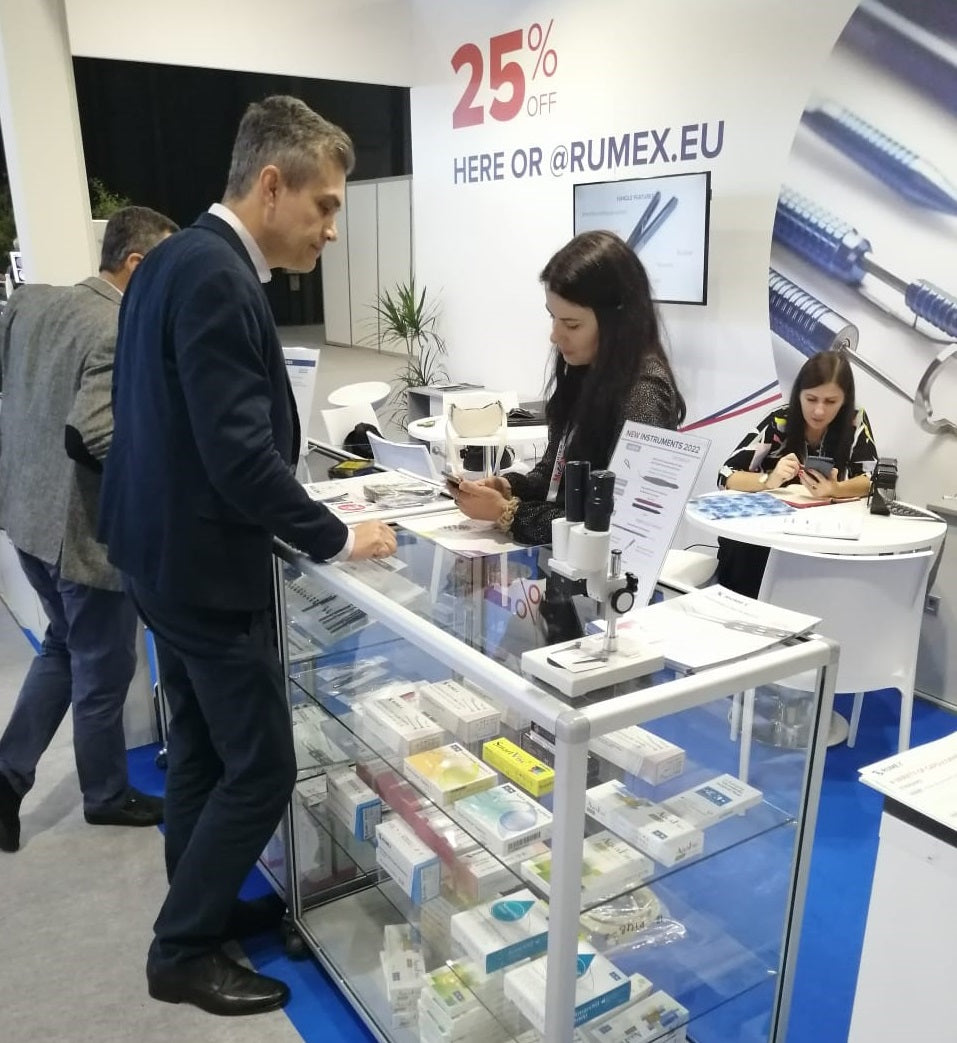


Welcome to ASCRS 2022!
We are happy to announce that RUMEX team takes part in annual ASCRS meeting.
Meet us in Washington, April 23-25, 2022. Walter. E. Washington Convention Centre, Booth #853
You are welcome to order RUMEX instruments in advance till April 11, 2022 and get your order ready packed at the show.
Your pre-order advantages:
- No queues
- No additional taxes
- Guaranteed stock
How to order:
- Order and pay RUMEX instruments till April 11, 2022 via your sales manager or customer service department
- Your order will be collected and sent to the show
- You’ll get your order ready packed at the exhibition at RUMEX booth# 853
Test results on the RUMEX OVD SUPREME performance
Product description:
SUPREME is a dispersive viscoelastic solution (OVD) of low molecular weight, a highly purified grade of hydroxypropyl methylcellulose (HPMC) 2%, clear, isotonic, sterile, non-inflammatory and non-pyrogenic in nature. It is used for intraocular injection during anterior segment surgery. The molecular weight is 86 000 Da. Viscosity is 3000 – 4500 cSt at 27° С.
Objectives:
1. To check whether the RUMEX OVD SUPREME can be completely removed from the anterior chamber after the phacoemulsification procedure is complete;
2. To check whether the intraocular pressure spikes take place during the postoperative period.
Patients and methods:
Forty-two eyes of 42 patients (71.6±7.7 years; 28 women and 14 men) that had grades 0 to 4 Emery–Little nuclear or cortical cataract without any other abnormalities were studied. The number of cases with glaucoma – 5. Phacoemulsification was performed using Alcon Infiniti. Intraocular pressure was measured with Alcon Infiniti. Huviz and Maklakov tonometer.
Table 1. The results of the IOP measurements with the tonometer before the surgery 1, 7 and 30 days after the surgery.
|
Intraocular pressure measurements, mmHg |
|||
Before the surgery |
1 day after the surgery |
7 days after the surgery |
30 days after the surgery |
|
1 |
16 |
14 |
15 |
15 |
2 |
18 |
16 |
16 |
16 |
3 |
18 |
17 |
17 |
17 |
4 |
21 |
18 |
18 |
18 |
5 |
22 |
20 |
20 |
20 |
6 |
20 |
18 |
18 |
18 |
7 |
21 |
20 |
20 |
20 |
8 |
24 |
27(+3mm) |
21 |
21 |
9 |
24 |
24 |
22 |
21 |
10 |
18 |
18 |
18 |
18 |
11 |
20 |
16 |
18 |
18 |
12 |
21 |
20 |
20 |
20 |
13 |
23 |
25(+2mm) |
21 |
21 |
14 |
25 |
21 |
21 |
21 |
15 |
20 |
24(+4mm) |
19 |
19 |
16 |
26 |
28(+2mm) |
22 |
22 |
17 |
18 |
22(+4mm) |
17 |
17 |
18 |
18 |
17 |
17 |
17 |
19 |
19 |
17 |
18 |
18 |
20 |
17 |
16 |
16 |
17 |
21 |
21 |
18 |
18 |
19 |
22 |
20 |
18 |
18 |
18 |
23 |
19 |
17 |
17 |
16 |
24 |
19 |
18 |
17 |
17 |
25 |
23 |
21 |
20 |
20 |
26 |
16 |
16 |
16 |
16 |
27 |
19 |
22(+3mm) |
18 |
19 |
28 |
20 |
20 |
20 |
20 |
29 |
20 |
18 |
18 |
18 |
30 |
21 |
19 |
19 |
19 |
31 |
22 |
20 |
20 |
20 |
32 |
22 |
21 |
20 |
20 |
33 |
19 |
17 |
17 |
17 |
34 |
18 |
24(+6mm) |
18 |
18 |
35 |
20 |
20 |
20 |
20 |
36 |
21 |
19 |
19 |
19 |
37 |
19 |
17 |
17 |
16 |
38 |
25 |
21 |
21 |
20 |
39 |
25 |
20 |
20 |
21 |
40 |
21 |
18 |
18 |
18 |
41 |
23 |
25(+2mm) |
22 |
22 |
42 |
18 |
16 |
16 |
16 |
Results:
A study reported (See Table 1) 19% (8 patients) incidence of IOP spikes (2-6 mmHg) 24 hours following phacoemulsification and IOL implantation. IOP level was stabilized 7 days following the cataract surgery and did not influence the postoperative eye condition and visual function of the patients. The IOP spike of 5 mmHg was observed in 1 patient diagnosed with glaucoma. The IOP spike of 2 mmHg was observed in 3 patients, all 3 diagnosed with glaucoma. The IOP spike of 3 mmHg was observed in 2 patients, one of them diagnosed with glaucoma. The IOP spike of 4 mmHg was observed in 2 patients, both had the grade 4 cataract.
The patients with IOP spikes of 4 to 6 mmHg were treated with antihypertensive drugs. The IOP was stabilized 7 days following the cataract surgery.
For most patients, the IOP decreased by 1- 4 mmHg one day postoperative. The most common complication in patients was cornea edema related to the high cataract grade, which has gone by the period up to 7 days postoperative.
To measure the rate at which residual OVD is removed from the anterior chamber, the amount of the BSS used for irrigation-aspiration after the IOL implantation was analyzed.
Table 2. The amount of BSS used for RUMEX OVD Supreme removal from the anterior chamber of the eye.
Patients |
The amount of BSS, ml |
Patients |
The amount of BSS, ml |
Patients |
The amount of BSS, ml |
1 |
12±1 |
15 |
10±1 |
29 |
10±1 |
2 |
15±1 |
16 |
12±1 |
30 |
10±1 |
3 |
9±1 |
17 |
12±1 |
31 |
9±1 |
4 |
10±1 |
18 |
11±1 |
32 |
12±1 |
5 |
13±1 |
19 |
12±1 |
33 |
13±1 |
6 |
12±1 |
20 |
13±1 |
34 |
13±1 |
7 |
10±1 |
21 |
14±1 |
35 |
12±1 |
8 |
12±1 |
22 |
11±1 |
36 |
12±1 |
9 |
11±1 |
23 |
12±1 |
37 |
11±1 |
10 |
11±1 |
24 |
13±1 |
38 |
10±1 |
11 |
12±1 |
25 |
11±1 |
39 |
12±1 |
12 |
11±1 |
26 |
12±1 |
40 |
12±1 |
13 |
13±1 |
27 |
13±1 |
41 |
11±1 |
14 |
11±1 |
28 |
14±1 |
42 |
13±1 |
According to the date in table 2, the average amount of BSS used for the complete removal of SUPREME from the anterior chamber of the eye is 12,2 +-1 ml.
Table 3. The data with the time spent for a surgery.
Patients |
Time spent for a surgery, min |
Patients |
Time spent for a surgery, min |
Patients |
Time spent for a surgery, min |
1 |
10 |
15 |
6 |
29 |
8 |
2 |
9 |
16 |
7 |
30 |
9 |
3 |
7 |
17 |
8 |
31 |
9 |
4 |
8 |
18 |
8 |
32 |
8 |
5 |
7 |
19 |
9 |
33 |
10 |
6 |
6 |
20 |
8 |
34 |
9 |
7 |
8 |
21 |
6 |
35 |
6 |
8 |
2 |
22 |
7 |
36 |
8 |
9 |
7 |
23 |
7 |
37 |
10 |
10 |
8 |
24 |
9 |
38 |
8 |
11 |
8 |
25 |
8 |
39 |
7 |
12 |
9 |
26 |
9 |
40 |
10 |
13 |
13 |
27 |
9 |
41 |
15 |
14 |
7 |
28 |
7 |
42 |
10 |
According to the test results showed in table 3, the average time needed for the RUMEX OVD Supreme removal from the anterior chamber is 8,5 min.
The fact of the complete removal of the OVD is checked with the help of the microscope during the surgery and after the surgery is complete.
Thus, RUMEX OVD Supreme is completely removed from the anterior chamber with the help of Balanced Salt Solution.
Conclusion:
The viscoelastic device SUPREME manufactured by RUMEX international LTD, United Kingdom, is completely removed from the anterior chamber of the eye with the Balanced Salt Solution.
The mild IOP spikes take place in patience 24 hours following the cataract surgery.
The IOP stabilizes within one week after the surgery.
Chief Extraordinary ophthalmologist
of Ministry of Health in Novgorod region D.V. Gatilov
14.02.2018
DMEK trends. Scooba vs Injection.
We would like to update conversation with Dr. Abdullayev, MD, MBA, CEBT, Manager of Clinical Development and Innovations at Lions Eye Institute for Transplant & Research, Inc., USA.
Today the discussion will be focused on DMEK issues and instruments developed for this technique.
- Dr. Abdullayev, please let us know more about the DMEK trends?
Basically Scooba method is the first method used to separate the Descemet's membrane and endothelium from the stroma. The Descemet's membrane is pulled from the stroma by use of special forceps.
In 2016 I introduced a BLISTER method for separation of the Descemet's membrane and endothelium from the stroma, the method of Optisol GS injection between the posterior stroma and Descement’s membrane and their subsequent separation. Nowadays, Trypan Blue, instead of Optisol GS, is used for injection and separation of the Descemet’s membrane, allowing instant staining of the graft.
BLISTER method is gaining interest among professionals who prepare DMEK grafts. But since Scooba method is still performed, I’ve recently developed Abdullayev DMEK Grasping Forceps, 4-261S in cooperation with RUMEX International, which is not only suitable for the Scooba method, but also for completing the separation when using the injection method, in cases when it’s necessary.
The instrument has the following advantages:
- Improved angle between grasping platform; the rest of the forceps allows relaxing hand position and more control when in use
- Horizontal thin grasping platform providing more stability in membrane holding during separation
- Ergonomic design
See lessAdvanced marking of DMEK and DSAEK grafts.
We keep on communicating with Dr. Abdullayev, MD, MBA, CEBT, Manager of Clinical Development and Innovations at Lions Eye Institute for Transplant & Research, Inc., USA.
Today we will go deeper into graft management and discuss graft marking and tools designed to facilitate this process.
- Dr. Abdullayev, please tell what problems you face during graft preparation. Are they common to Eye Banks?
Preparation of the grafts for endothelial keratoplasty such as DSAEK and DMEK is a complicated and delicate process. In the USA in 99.9% of cases a procedure takes place in the Eye Banks. There are several problems Eye Banks face during preparation - inconsistency of graft thickness, cells damage, inadequate markings placement. One of the main problems is associated with the ink marking (determining the graft orientation inside the eye) smearing and running off from the surface of the graft. Most grafts are prepared the day before the procedure and arrive to the operating theater at the day of surgery. If the mark runs off, it becomes difficult to follow it. In addition, the marks are too wide (for example, the letter S), whereas the ink is toxic for endothelial cells.
- Please advise the other methods of marking.
There are several methods of marking but most common in USA is “S” mark on the stromal surface of the DMEK and DSAEK grafts. Placing “S” mark is a complicated procedure (especially when DMEK graft needs to be marked) and requires additional manipulation with isolated graft of 10 microns, which can lead to endothelial cells loss, Descemet’s membrane damage, extended exposure of the endothelial cells to air and other disadvantages. I’ve developed, in cooperation with RUMEX International, a new instrument and technique for more advanced marking of the DMEK and DSAEK grafts which eliminates and reduces disadvantages, associated with “S” marking.
This novel Abdullayev I&II marker (for DMEK /DSAEK grafts), 3-024T is manufactured by RUMEX International and has the following advantages:
- I&II marks do not interfere with vision
- More stable staining
- Allows to apply 1.5mm straight I&II marks at the very edge of the graft
- No additional tissue manipulation (no punch holes, no folding or unfolding of the graft)
- Ergonomic design
- Do not rust – made of titanium
- Saves time during graft preparation
- Reusable and easy sterilizable
- Are there any other inventions for graft marking?
I’ve also developed, in cooperation with RUMEX International, the following markers for graft preparation:
Scleral Marker for Keratoplasty, Double-Ended 3-0230 (16.00 mm\16.50 mm Diameters)
It improves scleral rim trimming process for corneas with large scleral rim prior to microkeratome processing and eliminates additional measurement.
The other one is Abdullayev Corneal Marker with Central Dot for Keratoplasty, Double Ended 3-0231 (10.00 mm and 11.00 mm Diameters)
It has the following advantages:
- Allows quick placement of the central dot
- Improves centration of the cornea during DSAEK microkeratome preparation
- Improves centration of the donor corneas when placed on the donor punch
See lessNew trends in implantation of DSAEK grafts in the USA.
We continue conversation with Dr. Abdullayev, MD, MBA, CEBT, Manager of Clinical Development and Innovations at Lions Eye Institute for Transplant & Research, Inc., USA.
This column will be dedicated to graft management.
- Dr. Abdullayev, please share the trends in the implantation of DSAEK grafts in the USA
There are several trends and the main is to decrease the graft thickness and make DSAEK procedure less invasive (decrease an insertion wound size). I am proud that we are leaders, and I personally pioneered developing DSAEK graft thinning and less invasive insertion techniques. To compare, the average thickness of DMEK grafts is only 10 microns. In case of DSAEK grafts, the stroma plays a role of a carrier of Descemet’s membrane with endothelial cells but makes graft thicker. In 2006, when we started DSAEK, its thickness was 170 microns and thicker. In 2012, I introduced one of the first techniques to prepare DSAEK grafts with thickness 100 microns and thinner. In 2014 I introduced the first technique to prepare DSAEK grafts with thickness 40-60 microns which became clinically advanced (same visual recovery as after DMEK) compared to DSAEK grafts with thickness 100 microns and thicker. Since 2006 multiple DSAEK grafts delivery instruments were developed -forceps, Busin glide, EndoSerter, Endoglide which required delivery of the graft by touch, large (4-5mm) incision, anterior chamber maintainer, multiple closing sutures and had other disadvantages. These listed disadvantages are eliminated with introduction of our LEITR DSAEK 3.0.
- Please advise forceps to hold such a thin graft.
Many forceps that are available on the market have 150 microns distance between the branches and were designed for DSAEK grafts with thickness 100 microns and thicker; when thin(less than 100 microns) the graft often slides off the jaws of such forceps. This problem is widespread, so recently we designed and together with Rumex International have manufactured special forceps for graft holding with 120 microns distance between the branches.
It’s Lambright-Abdullayev Ultrathin DSAEK Donor Grasping Forceps, 4-254S, which allows to safely grasp DSAEK grafts with thickness 70 microns and thinner. A wave-shaped serration of grasping platforms allows for non-slip insertion and minimizes the risk of tissue adherence when the forceps is removed.
See lessThe trends of posterior keratoplasty. LEITR DSAEK 3.0.
RUMEX International Co developed a set of instruments for corneal transplantation in cooperation with Dr. Abdullayev, MD, MBA, CEBT, Manager of Clinical Development and Innovations at Lions Eye Institute for Transplant & Research, Inc., USA.
Dr. Abdullayev has been involved in Eye Banking community since 1996 and has many scientific publications on new techniques and procedures of Eye Banking, corneal transplantation and preservation.
We’re running a column to share his rich experience and knowledge. This information will be interesting not only for doctors, specializing in corneal surgery, but to everyone who is concerned with the subject of transplantation.
- Dr. Abdullayev, please tell us about the trends of posterior keratoplasty?
Nowadays, the amount of penetrating keratoplasty decreases while the amount of posterior endothelial keratoplasty increases.
According to Annual report of Eye Bank Association of America, the number of penetrating grafts performed in the U.S. using intermediate-term preservation increased slightly this year from 17,347 to 17,409 but had decreased in each of the previous 13 years from a high of 42,063 in 2005 to a low of 17,347 in 2018. In the past 10 years, EK procedures increased from 1,308 in 2010 to 30,650 in 2019. The number of corneas used domestically for EK has increased every year since tracking started in 2005 and surpassed PK in 2012. EK has been the most common keratoplasty procedure performed in the U.S. since 2012. The number of EK procedures (which includes both DSAEK and DMEK) increased 1% in 2019 to 30,650 from 30,336 in 2018.
- Not so long ago you’ve invented the innovative DSAEK graft injection method. Please let us know more about it.
Forceps method and pull in methods (require additional unloading forceps) are current techniques in the world for delivery of DSAEK graft into recipient eye. They cannot be preloaded in advance, require a large, usually between 4 to 5 mm incision, anterior chamber maintainer, resulting in significant endothelial cells loss up to 30-40%, and require at least 3 closing sutures. My innovative DSAEK graft injection method is a LEITR DSAEK 3.0 (just presented at the Cornea & Eye Banking Forum 2020 on November 7th). It is a novel carrier and technique for delivering by injection of an advance eye bank preloaded DSAEK grafts through smaller wound.
Primary advantages of LEITR DSAEK 3.0 are:
- Less invasive. Requires small 3.2-3.4mm incision (only 1 closing suture is required)
- Allows delivery of DSAEK grafts into anterior chamber by fluid injection - no-touch delivery
- Closed system advantages:
- no anterior chamber maintainer is required
- deepens AC chamber
- eases graft opening
- Low, only up to 5% endothelial cells loss during transport and transplant
See lessMeet RUMEX Flushing System for microinvasive tips!
For manufacturers of ophthalmic tools the working tip of vitreoretinal and microinvasive instruments is among the most difficult jobs to perform. Not only it has to be reliable, precise, sharp, etc. despite its tiny size; we also have to make sure that the tip can be properly cleaned so that patients are not at risk of contamination, and the instrument is not at risk of mechanical damage, especially of breaking apart in the posterior segment of an eye.
The most frequent reason for tips sticking or even fracturing is blood and intraocular fluid remaining after a surgery and baked inside the tube during sterilization, blocking the inner mechanism of the instrument. To avoid this, you can either dispose of a single use instrument after each surgery, or sterilizing staff has to perfectly flush the tip inside before it is exposed to high temperature, i.e. before sterilization.
Again, there are two principal approaches to flushing. Our traditional and favorite solution is a two-piece instrument; its handle is detachable and cleaned separately, whereas the tip is flushable with a syringe through an adaptor supplied free of charge. An additional benefit of this approach is an opportunity to save by purchasing fewer universal handles than tips; typically, our customers buy two to three tips per handle. Although this option, preferred by the majority of our customers, is the best from flushing point of view, a considerable part of surgeons globally requested for one-piece instruments to skip assembling step.
Meeting customers’ wishes, we have developed RUMEX flushing system to solve the one-piece instrument tip cleaning problem.
The main component of the system is a side port for flushing that allows cleaning the tube with the zero stress to the jaws – as flushing liquid streams inside the tool towards the most delicate tip of the instrument tenderly washing it inside. Another component is a flushing cannula provided for free with every instrument.
Compare RUMEX solution with a solution of an A-brand, where water is provided from the distal part,
its pressure is high enough to damage delicate tips, but not intensive enough to guarantee flushing the tube through its entire length. Vacuum and bi-directional flow of water associated with this style of cleaning can bring a potential risk too.
According to our customers, RUMEX flushing system “provides [them] with a fast, convenient and efficient way to take care of the tool without its disassembling“. “The easy cleaning procedure helps eliminate protein residuals inside the tube thus reducing risk for the patients and increase the service life on an instrument considerably”.
See lessSign up for RUMEX news
100% free, Unsubscribe any time!